The Problem:
The measurement of pH in the ocean is extremely important and indispensable in providing an understanding of the ocean’s health. Biofouling has long been considered a limiting factor for oceanographic studies and has been recognized as one of the main obstacles to autonomous environmental monitoring in coastal and ocean environments, as the rapid covering of bio growth on sensors disrupts the long-term quality of the measurements.
Solutions:
Many potential solutions to improve biofouling resistance for long-term deployments have been proposed (Manov et al.) but none are universally suitable.
- The most common approach for biofouling protection on oceanographic sensors is the use of mechanical devices such as wipers or scrapers. However, removing all bio growth is usually difficult due to the surface contours and fragility of the sensor (Delgado et al.).
- An alternative to the wipers is the generation of biocides to kill any organisms on the sensor. Previously the biocide antifouling market was dominated by tributyltin (TBT) biocide-based coatings. The TBT slowly leaches out into the marine environment removing any adhered substances when hydrolysing. However, it was proven to be highly toxic towards non-target organisms and showed very harmful environmental effects (Donnelly et al.).
- Today the most commonly used biocide system is the copper corrosion mechanism, where as the copper corrodes in seawater, oxidized Cu2+ based molecules are released into the water which interfere with the enzymes on cell membranes, avoiding cellular division. Copper-based antifouling paint can be classified into two groups; the first are slow-release films, releasing cuprous oxide into the surrounding environment by leaching. The second are ablative antifouling paints that have a continuously toxic surface. Even though copper is an efficient antifoulant, it is not effective against all the fouling species such as various algae, and furthermore, for a pH sensor, the effect the leached copper molecules have on the pH of the localised area around the sensor may lead to erroneous pH measurements.
- Another common antifouling system is electro-chlorination, which uses an electrolytic cell to transform the salt of seawater (NaCl) into sodium hypochlorite (NaOCl) through an electrolysis reaction. NaOCl acts as a disinfectant to the organic material and is a very effective antifoulant. Sodium hypochlorite solution has a 0.5 – 2 % concentration of chlorine, which is dilute enough to be environmentally safe. Moreover, the reaction that leads to the chlorine formation is completely reversible, so NaOCl transforms back to NaCl, leaving no remaining hypochlorite (Casson).
None of the antifouling methods described above are really fit for purpose for today’s pH sensors; they either change the pH of the surrounding area leading to erroneous results, or require rigorous wiping which can damage the fragile pH sensors.
ANB’s Solution:
Biofouling was a key consideration for us when designing our sensor, so we went for a two-prong approach to ensure our solution was biofouling-resistant.
- Firstly, our sensor is all solid-state and robust, tough enough to allow rigorous wiping. In fact, the only maintenance required by the sensor is an abrasion of the sensing electrodes on the surface, thus, a wiper could benefit the long-term performance of the sensor.
- Secondly, the electrochemical method utilized produces a low concentration of hydrogen peroxide (H2O2) which inhibits the formation of bacterial biofilm on the sensing element. This is effective against a broad range of bacteria, including many of those that have become resistant to chlorine-based chemicals. The low concentration of hydrogen peroxide generated local to the surface of the sensor is soon diluted and broken down so as to be non-hazardous to the external environment, but extremely effective for biofouling purposes.
To study and prove the biofouling resistance of our pH sensor it was deployed in the OBSEA platform (expandable seafloor observatory) in the Mediterranean Sea, in collaboration with Universitat Politecnica de Catalunya (UPC) (Jerico Next Funding), where biofouling is prevalent.
The main objective of the OBSEA platform is to provide relatively low-cost, continuous, remote, and long-term monitoring of the local coastal ecosystem, allowing real-time communication with the devices, as well as providing accessible infrastructure for the development of new marine sensors. The OBSEA platform was the perfect location for us to study and understand the biofouling resistance of our calibration-free pH sensor, since that Mediterranean region is recognized as a hot spot for climatic changes with significant seasonal variation in weather and biodiversity, making biofouling prevalent.
ANB’s pH sensor was deployed for 6 weeks on the coastal observing station at 20m depth. The results obtained were outstanding, as, after 6 weeks deployment, the transducer (the active sensing part) showed no evidence of biofouling (Figure 1) despite the sensor housing being covered in bio growth (Figure 2).
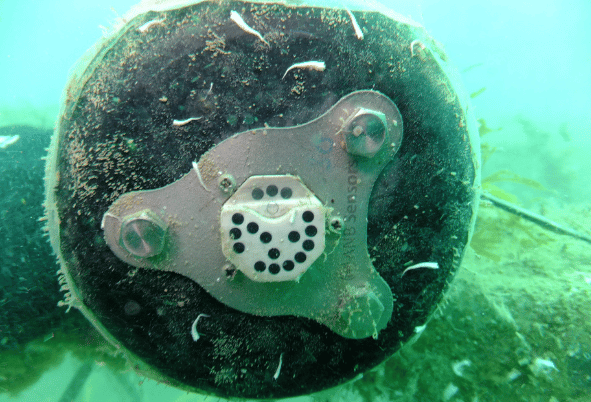
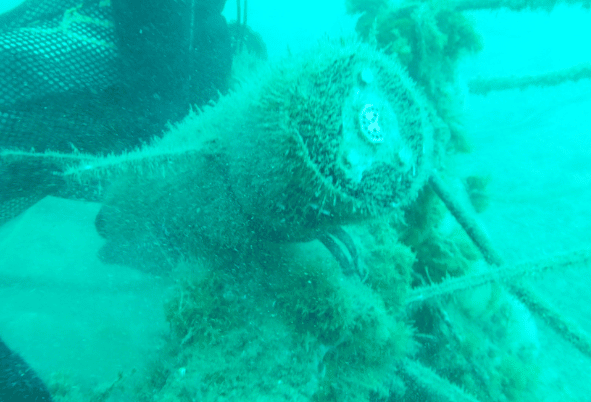
As a control, another sensor was also deployed in the same location for 3 weeks but left in sleep mode. As the sensor was not sampling, no hydrogen peroxide was formed, and therefore the biofouling was not inhibited, and clear bio growth can be seen on the surface of the sensing part (Figure 3). After 3 weeks this sensor was abraded underwater, removing all bio growth, and refreshing the sensor ready for deployment (Figure 4).
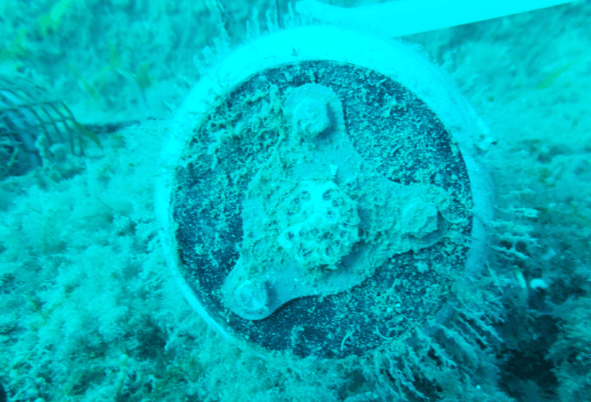
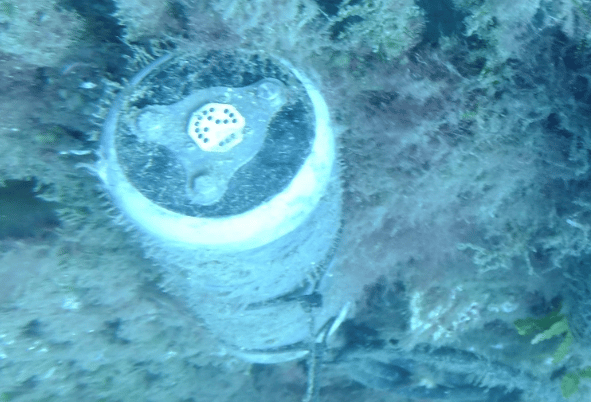
Conclusions:
These tests confirmed that ANB’s pH sensor can be autonomously operated in an environment where biofouling is prevalent without detriment to the results.
ANB’s pH sensor demonstrates a big advance in water quality monitoring technology. Its calibration-free feature along with its robustness and bio-growth resistance make it the go-to choice for measuring pH in marine environments.